Electromechanical processes during the start of induction-type magnetic levitation
DOI:
https://doi.org/10.20998/2074-272X.2025.3.01Keywords:
magnetic levitation of induction type, mathematical model, experimental test, starting electromechanical characteristics, oscillatory electromechanical processAbstract
Purpose. A study of induction-type magnetic levitation by determining the electromechanical processes that occur when a stationary inductor is connected to an alternating voltage source and the levitation of an anchor made in the form of a multi-turn short-circuited winding with an attached load. Methodology. Using a mathematical model describing an inductor and an anchor with concentrated parameters, solutions are presented for equations describing the interconnected electrical, magnetic, mechanical and thermal processes that occur in induction-type magnetic levitation. Results. The influence of the frequency of the alternating current source on the electromechanical processes of levitation, which occur at different parameters of the anchor, is established. Due to the phase delay of the induced anchor current in relation to the inductor current, an electrodynamic force directed downwards arises at certain moments of their period. The total force acting on the anchor, due to the electrodynamic component, is of an alternating nature with a predominance of the positive, upwardly directed component, which causes pulsations of the anchor speed. Originality. The force acting on the anchor due to the electrodynamic component is of an alternating nature with the positive component directed upwards dominating. The resulting oscillatory damping mechanical process occurs with an increase in the oscillation period and a decrease in its amplitude. Practical value. It has been established that the maximum value of the lifting force acting on the anchor is achieved at an alternating current frequency in the range from 75 to 125 Hz, and the highest value of the steady-state levitation height is realized for an anchor similar to an inductor at a frequency of 75 Hz. References 37, figures 6.
References
Han H.-S., Kim D.-S. Magnetic Levitation. Maglev Technology and Applications. Springer Publ., 2016. 247 р. doi https://doi.org/10.1007/978-94-017-7524-3.
Ramirez-Neria M., Gonzalez-Sierra J., Garcia-Antonio J.L., Garcia-Antonio J.A., Ramirez-Neria E. On the Sliding Mode Control of a Magnetic Levitation System Case: Thomson’s Jumping Ring. Proceedings of the International Conference of Control, Dynamic Systems, and Robotics, 2014, paper no. 105. 8 р.
Poletkin K.V., Asadollahbaik A., Kampmann R., Korvink J.G. Levitating Micro-Actuators: A Review. Actuators, 2018, vol. 7, no. 2, art. no. 17. doi: https://doi.org/10.3390/act7020017.
Carneiro P., Soares dos Santos M.P., Rodrigues A., Ferreira J.A.F., Simões J.A.O., Marques A.T., Kholkin A.L. Electromagnetic energy harvesting using magnetic levitation architectures: A review. Applied Energy, 2020, vol. 260, art. no. 114191. doi: https://doi.org/10.1016/j.apenergy.2019.114191.
Poletkin K. On the Static Pull-In of Tilting Actuation in Electromagnetically Levitating Hybrid Micro-Actuator: Theory and Experiment. Actuators, 2021, vol. 10, no. 10, art. no. 256. doi: https://doi.org/10.3390/act10100256.
Zhou B., Dai C., Liu Z., Yu J., Liu H., Zhang B. Design and optimization of H-type asymmetric magnetic suspension vibration absorber for ships. Journal of Vibration and Control, 2025, vol. 31, no. 3–4, pp. 344-354. doi: https://doi.org/10.1177/10775463241226840.
Liao H., Yuan H., Xie J. High-Precision Composite Control of Driving Current for Non-Contact Annular Electromagnetic Stabilized Spacecraft Subject to Multiple Disturbances. Aerospace, 2024, vol. 11, no. 8, art. no. 627. doi: https://doi.org/10.3390/aerospace11080627.
Han W., Cai Y., Han W., Yin Z., Yu C. Review on Active Vibration Control Method of Magnetically Suspended System. IEEE Access, 2023, vol. 11, pp. 108117-108125. doi: https://doi.org/10.1109/ACCESS.2023.3317333.
Fang J., Zheng S., Han B. Attitude Sensing and Dynamic Decoupling Based on Active Magnetic Bearing of MSDGCMG. IEEE Transactions on Instrumentation and Measurement, 2012, vol. 61, no. 2, pp. 338-348. doi: https://doi.org/10.1109/TIM.2011.2164289.
Cai Y., Yu C., Ren Y., Wang W., Yin Z., Xia C. High Precision Attitude-Rate Measurement of Magnetically Suspended Control and Sensing Gyroscope Using Variational Mode Decomposition and Wavelet Transform. IEEE Sensors Journal, 2022, vol. 22, no. 2, pp. 1188-1198. doi: https://doi.org/10.1109/JSEN.2021.3131994.
Dai C., Liu Z., Wang Y., Lin X., Liu H., Zhou B. Design and Optimization of a New Type of Magnetic Suspension Vibration Absorber for Marine Engineering. Journal of Marine Science and Engineering, 2023, vol. 11, no. 11, art. no. 2070. doi: https://doi.org/10.3390/jmse11112070.
Li A.J., Zhou B.M., Jiang C.D., Liu D.Z., Wang E.H. Frequency-doubling displacement disturbance suppression of active magnetic bearing based on repetitive control. 2024 IEEE 10th International Power Electronics and Motion Control Conference (IPEMC2024-ECCE Asia), 2024, pp. 707-711. doi: https://doi.org/10.1109/IPEMC-ECCEAsia60879.2024.10567916.
Cao S., Niu P., Wang W., Zhao T., Liu Q., Bai J., Sheng S. Novel Magnetic Suspension Platform with Three Types of Magnetic Bearings for Mass Transfer. Energies, 2022, vol. 15, no. 15, art. no. 5691. doi: https://doi.org/10.3390/en15155691.
Bolyukh V.F., Vinnichenko A.I. Concept of an Induction-Dynamic Catapult for a Ballistic Laser Gravimeter. Measurement Techniques, 2014, vol. 56, no. 10, pp. 1098-1104. doi: https://doi.org/10.1007/s11018-014-0337-z.
Bolyukh V.F., Omel’chenko A.V., Vinnichenko A.I. Effect of Self-Seismic Oscillations of the Foundation on the Readout of a Ballistic Gravimeter with an Induction-Dynamic Catapult. Measurement Techniques, 2015, vol. 58, no. 2, pp. 137-142. doi: https://doi.org/10.1007/s11018-015-0675-5.
Shearwood C., Ho K.Y., Williams, C.B., Gong H. Development of a levitated micromotor for application as a gyroscope. Sensors and Actuators A: Physical, 2000, vol. 83, no. 1–3, pp. 85-92. doi: https://doi.org/10.1016/S0924-4247(00)00292-2.
Hatakenaka K., Hijikata W., Fujiwara T., Ohuchi K., Inoue Y. Prevention of thrombus formation in blood pump by mechanical circular orbital excitation of impeller in magnetically levitated centrifugal pump. Artificial Organs, 2023, vol. 47, no. 2, pp. 425-431. doi: https://doi.org/10.1111/aor.14443.
Tang J., Li C., Zhou J., Wu Z. Effects of mechanical interfaces on magnetic levitation systems and analysis of self-excited vibration mechanisms in coupled systems. Science China Technological Sciences, 2024, vol. 67, no. 12, pp. 3925-3939. doi: https://doi.org/10.1007/s11431-024-2776-8.
Shi H., Deng Z., Ke Z., Li Z., Zhang W. Linear permanent magnet electrodynamic suspension system: Dynamic characteristics, magnetic-mechanical coupling and filed test. Measurement, 2024, vol. 225, art. no. 113960. doi: https://doi.org/10.1016/j.measurement.2023.113960.
Wang X.G., Liu Q., Zhang Y.J., Chen H.H. Research on Characteristic of Electromagnetic Force of Magnetic Suspension Device with Large Air-Gap. Applied Mechanics and Materials, 2013, vol. 401-403, pp. 239-244. doi: https://doi.org/10.4028/www.scientific.net/AMM.401-403.239.
Abd. Aziz P.D., Li Q., Rodriguez E., Deng Z. A Preliminary Study on Electromagnetic Levitation Design Topology. 2020 IEEE International Conference on Applied Superconductivity and Electromagnetic Devices (ASEMD), 2020, pp. 1-2. doi: https://doi.org/10.1109/ASEMD49065.2020.9276251.
Bolyukh V.F., Shchukin I.S. Improving the Efficiency of a Linear Pulse Electromechanical Accelerator Due to Excitation by a Series of Pulses. 2020 IEEE KhPI Week on Advanced Technology (KhPIWeek), 2020, pp. 205-210. doi: https://doi.org/10.1109/KhPIWeek51551.2020.9250077.
Bolyukh V.F. Effect of electric conducting element on indicators of linear pulse electromechanical converter induction type. Technical Electrodynamics, 2020, no. 3, pp. 22-29. doi: https://doi.org/10.15407/techned2020.03.022.
Skubov D.Y., Indeitsev D.A., Udalov P.P., Popov I.A., Lukin A.V., Poletkin K.V. Nonlinear Dynamics of a Micromechanical Non-Contact Induction Suspension. Mechanics of Solids, 2023, vol. 58, no. 6, pp. 2011-2023. doi: https://doi.org/10.3103/S0025654423600307.
Rahman A., Mizuno T., Takasaki M., Ishino Y. An Equivalent Circuit Analysis and Suspension Characteristics of AC Magnetic Suspension Using Magnetic Resonant Coupling. Actuators, 2020, vol. 9, no. 3, art. no. 52. doi: https://doi.org/10.3390/act9030052.
Jeffery R.N., Amiri F. Thomson’s Jumping Ring Over a Long Coil. The Physics Teacher, 2018, vol. 56, no. 3, pp. 176-180. doi: https://doi.org/10.1119/1.5025301.
Tjossem P.J.H., Cornejo V. Measurements and mechanisms of Thomson’s jumping ring. American Journal of Physics, 2000, vol. 68, no. 3, pp. 238-244. doi: https://doi.org/10.1119/1.19407.
Donoso G., Ladera C.L. The naked toy model of a jumping ring. European Journal of Physics, 2014, vol. 35, no. 1, art. no. 015002. doi: https://doi.org/10.1088/0143-0807/35/1/015002.
Ng C. The Thomson Jumping Ring Experiment and Ideal Transformer. The Physics Teacher, 2022, vol. 60, no. 5, pp. 376-379. doi: https://doi.org/10.1119/5.0036490.
Ladera C.L., Donoso G. Unveiling the physics of the Thomson jumping ring. American Journal of Physics, 2015, vol. 83, no. 4, pp. 341-348. doi: https://doi.org/10.1119/1.4902891.
Tjossem P.J.H., Brost E.C. Optimizing Thomson’s jumping ring. American Journal of Physics, 2011, vol. 79, no. 4, pp. 353-358. doi: https://doi.org/10.1119/1.3531946.
Taweepong J., Thamaphat K., Limsuwan S. Jumping ring experiment: effect of temperature, non-magnetic material and applied current on the jump height. Procedia Engineering, 2012, vol. 32, pp. 982-988. doi: https://doi.org/10.1016/j.proeng.2012.02.042.
Bolyukh V.F., Shchukin I.S. Influence of an excitation source on the power indicators of a linear pulse electromechanical converter of induction type. Technical Electrodynamics, 2021, no. 3, pp. 28-36. doi: https://doi.org/10.15407/techned2021.03.028.
Bolyukh V.F., Katkov I.I. Influence of the Form of Pulse of Excitation on the Speed and Power Parameters of the Linear Pulse Electromechanical Converter of the Induction Type. Proceedings of the ASME 2019 International Mechanical Engineering Congress and Exposition. Volume 2B: Advanced Manufacturing, 2019, V02BT02A047. doi: https://doi.org/10.1115/IMECE2019-10388.
Bolyukh V.F., Kashansky Y.V., Schukin I.S. Features of excitation of a linear electromechanical converter of induction type from an AC source. Electrical Engineering & Electromechanics, 2021, no. 1, pp. 3-9. doi: https://doi.org/10.20998/2074-272X.2021.1.01.
Vaskovsky Yu.M., Chemeris V.T., Petrovsky V.P., Shats A.N. Physical modeling of the features of anchor acceleration in a pulse electromechanical converter of induction type. Technical Electrodynamics, 1986, no. 3, pp. 66-69.
Bolyukh V., Vinnichenko О., Neyezhmakov P., Omelchenko A. Reduction of auto seismic oscillations of the ballistic laser gravimeter on account of the excitation of the induction-dynamic catapult by a pulse packet. Ukrainian Metrological Journal, 2020, no. 3, pp. 3-11. doi: https://doi.org/10.24027/2306-7039.3.2020.216765.
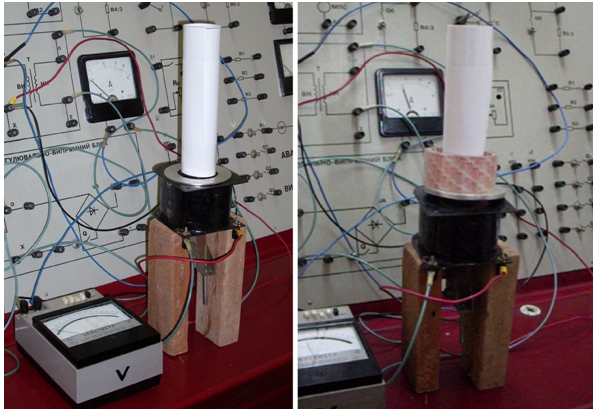
Downloads
Published
How to Cite
Issue
Section
License
Copyright (c) 2025 V. F. Bolyukh

This work is licensed under a Creative Commons Attribution-NonCommercial 4.0 International License.
Authors who publish with this journal agree to the following terms:
1. Authors retain copyright and grant the journal right of first publication with the work simultaneously licensed under a Creative Commons Attribution License that allows others to share the work with an acknowledgement of the work's authorship and initial publication in this journal.
2. Authors are able to enter into separate, additional contractual arrangements for the non-exclusive distribution of the journal's published version of the work (e.g., post it to an institutional repository or publish it in a book), with an acknowledgement of its initial publication in this journal.
3. Authors are permitted and encouraged to post their work online (e.g., in institutional repositories or on their website) prior to and during the submission process, as it can lead to productive exchanges, as well as earlier and greater citation of published work.