Distribution of volumes of plasma channels components between metal granules in working liquids
DOI:
https://doi.org/10.20998/2074-272X.2025.1.10Keywords:
plasma channels, spark, discharge current, statistical distributions, metal granules, erosion particlesAbstract
Introduction. Expanding the capabilities of a number of modern technologies and improving quality of their products require detailed spark and plasma erosion processes control in metal granules layers (MGL). Problem. Traditional measurement of exclusively electrical parameters of these processes, even in the case of multi-electrode systems, provides only a general vision, not allowing monitoring processes in individual plasma channels. Optical control methods make it possible to simultaneously have information about almost every plasma channel in the MGL. The aim of the article is to study the characteristic components of plasma channels arising as a result of the flow of discharge currents in the MGL and to establish the laws of distribution of their volumes and their ratios. Methodology. During the experiments, photographs of plasma channels resulting from the flow of discharge current pulses between Al granules immersed in distilled water were obtained. Using the specialized ToupView program, the volumes of equivalent ellipsoids of rotation, approximating the colored halos and white cores of the plasma channels were determined. Discrete distributions of the volumes of the halo and cores of plasma channels, as well as their ratios were constructed both with and without procedures for screening out «anomalous» results. The efficiency of approximation of discrete distributions obtained in practice by continuous theoretical distributions Weibull, Rosin-Rammler and log-normal was estimated. Results. It is shown that of all the considered theoretical distributions of halo and cores of plasma channels volumes, as well as their ratios, the most adequate is the log-normal one. Originality. For the first time distributions of volumes of halo and cores of plasma channels were studied and their comparative analysis with the size distributions of erosion particles and dimples on the surface of Al granules was given. Practical significance. Taking into account the new obtained results, a technique for constructing distributions of volumes of halo and cores of plasma channels and determining their parameters has been developed. References 53, figures 7, tables 5.
References
Gilchuk A., Monastyrsky G. «Core–shell» nanoparticles produced from Ti-Ni-Hf and Ti-Ni-Zr alloys by spark erosion method. Applied Nanoscience, 2023, vol. 13, no. 11, pp. 7145-7154. doi: https://doi.org/10.1007/s13204-023-02864-9.
Jin C.H., Si P.Z., Xiao X.F., Feng H., Wu Q., Ge H.L., Zhong M. Structure and magnetic properties of Cr/Cr2O3/CrO2 microspheres prepared by spark erosion and oxidation under high pressure of oxygen. Materials Letters, 2013. vol. 92, pp. 213-215. doi: https://doi.org/10.1016/j.matlet.2012.10.126.
Berkowitz A.E., Hansen M.F., Parker F.T., Vecchio K.S., Spada F.E., Lavernia E.J., Rodriguez R. Amorphous soft magnetic particles produced by spark erosion. Journal of Magnetism and Magnetic Materials, 2003, vol. 254-255, pp. 1-6. doi: https://doi.org/10.1016/S0304-8853(02)00932-0.
Aur S., Egami T., Berkowitz A.E., Walter J.L. Atomic Structure of Amorphous Particles Produced by Spark Erosion. Physical Review B, 1982, vol. 26, no. 12, pp. 6355-6361. doi: https://doi.org/10.1103/PhysRevB.26.6355.
Hong J.I., Parker F.T., Solomon V.C., Madras P., Smith D.J., Berkowitz A.E. Fabrication of spherical particles with mixed amorphous/crystalline nanostructured cores and insulating oxide shells. Journal of Materials Research, 2008, vol. 23, no. 06, pp. 1758-1763. doi: https://doi.org/10.1557/JMR.2008.0199.
Perekos A.E., Chernenko V.A., Bunyaev S.A., Zalutskiy V.P., Ruzhitskaya T.V., Boitsov O.F., Kakazei G.N. Structure and magnetic properties of highly dispersed Ni-Mn-Ga powders prepared by spark-erosion. Journal of Applied Physics, 2012, vol. 112, no. 9. art. no. 093909. doi: https://doi.org/10.1063/1.4764017.
Harrington T., McElfresh C., Vecchio K.S. Spark erosion as a high-throughput method for producing bimodal nanostructured 316L stainless steel powder, Powder Technology, 2018, vol. 328, pp. 156–166. doi: https://doi.org/10.1016/j.powtec.2018.01.012.
Wang W., Zhu F., Weng J., Xiao J., Lai W. Nanoparticle morphology in a granular Cu–Co alloy with giant magnetoresistance, Applied Physics Letters, 1998, vol. 72, no 9, pp. 1118–1120. doi: https://doi.org/10.1063/1.120942.
Berkowitz A.E., Walter J.L. Spark Erosion: A Method for Producing Rapidly Quenched Fine Powders, Journal of Materials Research. 1987, no 2. pp. 277–288. doi: https://doi.org/10.1557/JMR.1987.0277.
Shen B., Inoue A. Fabrication of large-size Fe-based glassy cores with good soft magnetic properties by spark plasma sintering, Journal of Materials Research, 2003, vol. 18, no 9, pp. 2115–2121. doi: https://doi.org/10.1557/jmr.2003.0297.
Youssef F.S., El-Banna H.A., Elzorba H.Y., Gabal A.M. Application of Some Nanoparticles in the Field of Veterinary Medicine, International Journal of Veterinary Science and Medicine, 2019, vol. 7, no 1. pp. 78-93. doi: https://doi.org/10.1080/23144599.2019.1691379.
Batsmanova L., Taran N., Konotop Ye., Kalenska S., Novytska N. Use of a Colloidal Solutions of Metal and Metal Oxide-Containing Nanoparticles as Fertilizer for Increasing Soybean Productivity, Journal of Central European Agriculture, 2020, no 2 (21), pp. 311–319. doi: https://doi.org/10.5513/JCEA01/21.2.2414.
Petrov O., Petrichenko S., Yushchishina A., Mitryasova O., Pohrebennyk V. Electrospark Method in Galvanic Wastewater Treatment for Heavy Metal Removal. Applied Sciences, 2020, vol. 10, no. 15, art. no. 5148. doi: https://doi.org/10.3390/app10155148.
Goncharuk V.V., Shcherba A.A., Zakharchenko S.N., Savluk O.S., Potapchenko N.G., Kosinova V.N. Disinfectant action of the volume electrospark discharges in water. Khimiia i tehnologiia vody, 1999, vol. 21, no. 3, pp. 328-336. (Rus).
Shydlovska N.A., Zakharchenko S.M., Zakharchenko M.F., Mazurenko I.L., Kulida M.A. Physical and Technical-economic Aspects of Modern Methods of Water Treatment for Thermal and Nuclear Power Engineering. Technical Electrodynamics, 2022, no. 4, pp. 69-77. (Ukr). doi: https://doi.org/10.15407/techned2022.04.069.
Zakharchenko S.N., Kondratenko I.P., Perekos A.E., Zalutsky V.P., Kozyrsky V.V., Lopatko K.G. Influence of discharge pulses duration in a layer of iron granules on the size and structurally-phase conditions of its electroerosion particles. Eastern-European Journal of Enterprise Technologies, 2012. vol. 6, no. 5 (60), pp. 66-72. (Rus).
Shydlovska N.A., Zakharchenko S.M., Cherkaskyi O.P. Physical Prerequisites of Construction of Mathematical Models of Electric Resistance of Plasma-erosive Loads. Technical Electrodynamics, 2017, no 2, pp. 5-12. (Ukr) doi: https://doi.org/10.15407/techned2017.02.005.
Shydlovska N.A., Zakharchenko S.M., Cherkassky O.P. The Analysis of Electromagnetic Processes in Output Circuit of the Generator of Discharge Pulses with Non-linear Model of Plasma-erosive Load at Change Their Parameters in Wide Ranges. Technical Electrodynamics, 2016, no. 1. pp. 87-95. (Rus). doi: https://doi.org/10.15407/techned2016.01.087.
Shydlovska N.A., Zakharchenko S.M., Cherkaskyi O.P. Parametric model of resistance of plasma-erosive load, adequate in the wide range of change of applied voltage. Technical Electrodynamics, 2017, no 3, pp. 3-12. (Ukr) doi: https://doi.org/10.15407/techned2017.03.003.
Shydlovskaya N.A., Zakharchenko S.N., Cherkasskyi A.P. Nonlinear-parametrical model of electrical resistance of current-carrying granulated mediums for a wide range of applied voltage. Technical Electrodynamics, 2014, no 6, pp. 3-17. (Rus).
Zakharchenkо S.M., Perekos А.O., Shydlovska N.A., Ustinov A.I., Bоytsоv О.F., Vоynаsh V.Z. Electrospark Dispersion of Metal Materials. I. Influence of Velocity of Flow of Operating Fluid on Dispersity of Powders. Metallofizika i Noveishie Tekhnologii, 2018, vol. 40, no. 3, pp. 339-357 (Rus). doi: https://doi.org/10.15407/mfint.40.03.0339.
Carrey J., Radousky H.B., Berkowitz A.E. Spark-eroded particles: influence of processing parameters. Journal of Applied Physics, 2004, vol. 95, no. 3, pp. 823-829. doi: https://doi.org/10.1063/1.1635973.
Suprunovska N.І., Shcherba М.А., Roziskulov S.S., Synytsyn V.K. Improving the dynamic characteristics of electric discharge installations, which are significantly distant from the spark-erosion load. Technical Electrodynamics, 2022, no 3, pp. 16-21. doi: https://doi.org/10.15407/techned2022.03.016.
Kornev I., Saprykin F., Lobanova G., Ushakov V., Preis S. Spark erosion in a metal spheres bed: Experimental study of the discharge stability and energy efficiency. Journal of Electrostatics, 2018, vol. 96, pp. 111-118. doi: https://doi.org/10.1016/j.elstat.2018.10.008.
Gnedenko B.V. Theory of Probability. London, Routledge, 1998. 520 p. doi: https://doi.org/10.1201/9780203718964.
Shydlovska N.A., Zakharchenko S.M., Zakharchenko M.F., Kulida M.A., Zakusilo S.A. Spectral and optic-metric methods of monitoring parameters of plasma channels caused by discharge currents between metals granules in working liquids. Electrical Engineering & Electromechanics, 2024, no. 6, pp. 72-83. doi: https://doi.org/10.20998/2074-272X.2024.6.10.
Kim C.J. Electromagnetic Radiation Behavior of Low-Voltage Arcing Fault. IEEE Transactions on Power Delivery, 2009, vol. 24, no. 1, pp. 416-423. doi: https://doi.org/10.1109/TPWRD.2008.2002873.
Kozioł M., Nagi Ł., Kunicki M., Urbaniec I. Radiation in the Optical and UHF Range Emitted by Partial Discharges. Energies, 2019, vol. 12, no. 22, art. no. 4334. doi: https://doi.org/10.3390/en12224334.
Kozioł M. Energy Distribution of Optical Radiation Emitted by Electrical Discharges in Insulating Liquids. Energies, 2020, vol. 13, no. 9, art. no. 2172. doi: https://doi.org/10.3390/en13092172.
Kohut A., Ludvigsson L., Meuller B.O., Deppert K., Messing M.E., Galbács G., Geretovszky Z. From plasma to nanoparticles: optical and particle emission of a spark discharge generator. Nanotechnology, 2017, vol. 28, no. 47, art. no. 475603. doi: https://doi.org/10.1088/1361-6528/aa8f84.
Korytchenko K.V., Essmann S., Markus D., Maas U., Poklonskii E.V. Numerical and Experimental Investigation of the Channel Expansion of a Low-Energy Spark in the Air. Combustion Science and Technology, 2019, vol. 191, no. 12, pp. 2136-2161. doi: https://doi.org/10.1080/00102202.2018.1548441.
Lo A., Cessou A., Lacour C., Lecordier B., Boubert P., Xu D., Laux C.O., Vervisch P. Streamer-to-spark transition initiated by a nanosecond overvoltage pulsed discharge in air. Plasma Sources Science and Technology, 2017, vol. 26, no. 4. art. no. 045012. doi: https://doi.org/10.1088/1361-6595/aa5c78.
Mylnikov D., Efimov A., Ivanov V. Measuring and optimization of energy transfer to the interelectrode gaps during the synthesis of nanoparticles in a spark discharge. Aerosol Science and Technology, 2019, vol. 53, no. 12, pp. 1393-1403. doi: https://doi.org/10.1080/02786826.2019.1665165.
Raizer Yu.P. Gas Discharge Physics. Berlin, Springer, 1991. 449 p.
Baranov M.I. A generalized physical principle of development of plasma channel of a high-voltage pulse spark discharge in a dielectric. Electrical Engineering & Electromechanics, 2024, no. 1, pp. 34-42. doi: https://doi.org/10.20998/2074-272X.2024.1.05.
Korytchenko K.V., Shypul O.V., Samoilenko D., Varshamova I.S., Lisniak А.A., Harbuz S.V., Ostapov K.M. Numerical simulation of gap length influence on energy deposition in spark discharge. Electrical Engineering & Electromechanics, 2021, no. 1, pp. 35-43. doi: https://doi.org/10.20998/2074-272X.2021.1.06.
ToupTek. Download. Available at: https://www.touptekphotonics.com/download/ (Accessed: 28 June 2024).
Mishra P., Pandey C., Singh U., Gupta A., Sahu C., Keshri A. Descriptive statistics and normality tests for statistical data. Annals of Cardiac Anaesthesia, 2019, vol. 22, no. 1, pp. 67-72. doi: https://doi.org/10.4103/aca.ACA_157_18.
Arya R., Antonisamy B., Kumar S. Sample Size Estimation in Prevalence Studies. The Indian Journal of Pediatrics, 2012, vol. 79, no. 11, pp. 1482-1488. doi: https://doi.org/10.1007/s12098-012-0763-3.
Akagawa S., Odagaki T. Geometrical percolation of hard-core ellipsoids of revolution in the continuum. Physical Review E, 2007, vol. 76, no. 5, art. no. 051402. doi: https://doi.org/10.1103/PhysRevE.76.051402.
Menzies N.A. An Efficient Estimator for the Expected Value of Sample Information. Medical Decision Making, 2016, vol. 36, no. 3, pp. 308-320. doi: https://doi.org/10.1177/0272989X15583495.
Wu Z., Yang X., Tu J., Chen X. Optimal consistency and consensus models for interval additive preference relations: A discrete distribution perspective. Journal of the Operational Research Society, 2020, vol. 71, no. 9, pp. 1479-1497. doi: https://doi.org/10.1080/01605682.2019.1621219.
Burshtynska H.V., Yidunov A.V. The study of various statistical criteria in the processing of false points in the process of determining the elements of mutual orientation. Geodesy, Cartography and Aerial Photography, 1984, no. 39, pp. 114-118. (Rus).
Adikaram K.K.L.B., Hussein M.A., Effenberger M., Becker T. Data Transformation Technique to Improve the Outlier Detection Power of Grubbs’ Test for Data Expected to Follow Linear Relation. Journal of Applied Mathematics, 2015, vol. 2015, art. no. 708948. doi: https://doi.org/10.1155/2015/708948.
Zhao Y., Lehman B., Ball R., Mosesian J., de Palma J.-F. Outlier detection rules for fault detection in solar photovoltaic arrays. 2013 Twenty-Eighth Annual IEEE Applied Power Electronics Conference and Exposition (APEC), 2013, pp. 2913-2920. doi: https://doi.org/10.1109/APEC.2013.6520712.
Barbato G., Barini E.M., Genta G., Levi R. Features and performance of some outlier detection methods. Journal of Applied Statistics, 2011, vol. 38, no. 10, pp. 2133-2149. doi: https://doi.org/10.1080/02664763.2010.545119.
Ventzel E.S. Theory of Probability. Moscow, Nauka Publ., 1969. 576 p. (Rus).
Shidlovska N.A., Zakharchenko S.M., Perekos A.O. Peculiarities of the diameter distributions obtained at submilisecond duration of discharge pulses spark-erosive aluminum particles and caverns on the surface of its granules. Technical Electrodynamics, 2021, no. 1, pp. 10-22. (Ukr). doi: https://doi.org/10.15407/techned2021.01.010.
Mitzenmacher M. A brief history of generative models for power law and lognormal distributions. Internet Mathematics, 2004, vol. 1, no. 2, pp. 226-251. doi: https://doi.org/10.1080/15427951.2004.10129088.
Cuff V., Lewis A., Miller S.J. The Weibull distribution and Benford’s law. Involve, a Journal of Mathematics, 2015, vol. 8, no. 5, pp. 859-874. doi: https://doi.org/10.2140/involve.2015.8.859.
Kumar R., Gopireddy S.R., Jana A.K., Patel C.M. Study of the discharge behavior of Rosin-Rammler particle-size distributions from hopper by discrete element method: A systematic analysis of mass flow rate, segregation and velocity profiles. Powder Technology, 2020, vol. 360, pp. 818-834. doi: https://doi.org/10.1016/j.powtec.2019.09.044.
Franke T.M., Ho T., Christie C.A. The Chi-Square Test: Often Used and More Often Misinterpreted. American Journal of Evaluation, 2012, vol. 33, no. 3, pp. 448-458. doi: https://doi.org/10.1177/1098214011426594.
Kucheriava I.M. Multiphysics processes at spark erosion treatment of conducting granules. Technical Electrodynamics, 2017, no. 5, pp. 32-38. (Rus). doi: https://doi.org/10.15407/techned2017.05.032.
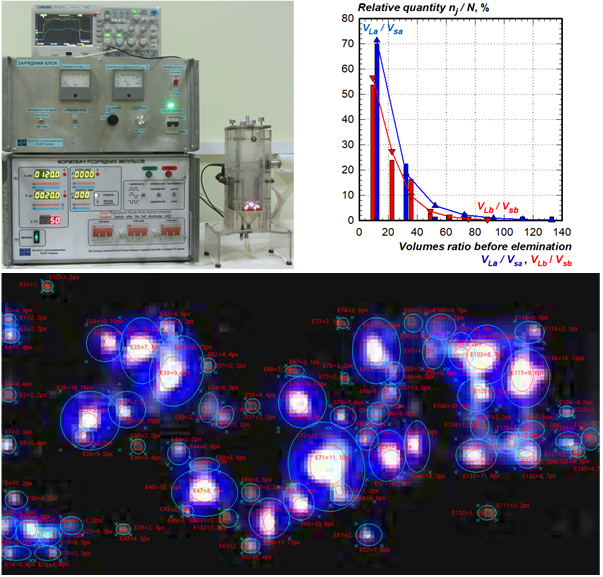
Downloads
Published
How to Cite
Issue
Section
License
Copyright (c) 2025 N. A. Shydlovska, S. M. Zakharchenko, M. F. Zakharchenko, M. A. Kulida, S. A. Zakusilo, R. A. Yakovenko

This work is licensed under a Creative Commons Attribution-NonCommercial 4.0 International License.
Authors who publish with this journal agree to the following terms:
1. Authors retain copyright and grant the journal right of first publication with the work simultaneously licensed under a Creative Commons Attribution License that allows others to share the work with an acknowledgement of the work's authorship and initial publication in this journal.
2. Authors are able to enter into separate, additional contractual arrangements for the non-exclusive distribution of the journal's published version of the work (e.g., post it to an institutional repository or publish it in a book), with an acknowledgement of its initial publication in this journal.
3. Authors are permitted and encouraged to post their work online (e.g., in institutional repositories or on their website) prior to and during the submission process, as it can lead to productive exchanges, as well as earlier and greater citation of published work.