Spectral and optic-metric methods of monitoring parameters of plasma channels caused by discharge currents between metals granules in working liquids
DOI:
https://doi.org/10.20998/2074-272X.2024.6.10Keywords:
discharge current, plasma channels, metal granules, optical emission spectrumAbstract
Introduction. Spark-erosion processing of metals and alloys granules in working liquids is the basis of a several technological processes. Efficiency of energy use in them and parameters of the resulting product largely depend on the accuracy of stabilization and regulation of pulse power in each plasma channel between the granules. To achieve this, until now only the voltage and current of the discharge pulses in the entire layer of granules have been controlled. Problem. The measurement methods, which are used, are not effective enough for monitoring the parameters of individual plasma channels and predicting the size distribution of eroded metals particles at the stage of their formation. The aim of the work is to develop a method for determining the volumes of components of plasma channels in layers of metals granules during their spark-erosion treatment to predict the size distribution of eroded metal particles at the stage of their formation, as well as to simplify the method of spectrometric analysis of the elemental composition of substances surrounding plasma channels for the operational prediction of the chemical composition of resulting products. Methodology. A series of experiments were carried out on spark-erosion processing of Al and Ag granules layers in distilled water. Using a digital camera, images of the plasma channels in them were obtained. Based on the theory of pulsed electrical breakdown of liquid dielectrics, an analysis of the components of plasma channels was carried out. Using the specialized ToupView program, the volumes of equivalent ellipsoids of rotation were determined, approximating the halos of colored radiation likely arising from streamers, as well as the spark cores of plasma channels emitting white light. The shades of the resulting radiation were studied for several metals and working liquids. The obtained data were compared with the known results of spectrometric studies for the same elements excited by similar mechanisms. Results. The theory of discharge-pulse systems for spark-erosion processing of granular conductive media has been developed in the direction of new methods for monitoring the parameters of discharge pulses and predicting the chemical composition and size distribution parameters of eroded metal particles at the stage of their production. An optic-metric method has been developed for determining the volumes of halos and cores of plasma channels. A simplified spectral method for determining the chemical composition of erosion particles based on the shade of the resulting radiation was proposed. Originality. The developed new optic-metric method makes it possible to obtain information about almost every plasma channel, which refines predictions of the size distribution of erosion particles. To implement the method, general-purpose hardware and specialized software that is freely available are used. The developed method of simplified spectral analysis of excited atoms makes it possible to make preliminary predictions of the chemical composition of the obtained erosion particles already at the stage of their formation without the use of expensive specialized equipment. Practical significance. The ratio of the volumes of halos and cores of plasma channels between Al and Ag granules in distilled water was measured. An analysis of the emission spectra of plasma channel halos between Al, Ag and Cu granules in distilled water, Fe in ethyl alcohol, Ni-Mn-Ga and Ti-Zr-Ni alloys in liquid nitrogen, and Ti-Zr-Ni in liquid argon was carried out. Based on spectrometry data, the resulting shades of these radiations were substantiated and their description in the RGB system is given. References 56, table 1, figures 4.
References
Ochin P., Gilchuk A.V., Monastyrsky G.E., Koval Y., Shcherba A.A., Zaharchenko S.N. Martensitic Transformation in Spark Plasma Sintered Compacts of Ni-Mn-Ga Powders Prepared by Spark Erosion Method in Cryogenic Liquids. Materials Science Forum, 2013, vol. 738-739, pp. 451-455. doi: https://doi.org/10.4028/www.scientific.net/MSF.738-739.451.
Monastyrsky G. Nanoparticles formation mechanisms through the spark erosion of alloys in cryogenic liquids. Nanoscale Research Letters, 2015, vol. 10, no. 1, art. no. 503. doi: https://doi.org/10.1186/s11671-015-1212-9.
Aur S., Egami T., Berkowitz A.E., Walter J.L. Atomic Structure of Amorphous Particles Produced by Spark Erosion. Physical Review B, 1982, vol. 26, no. 12, pp. 6355-6361. doi: https://doi.org/10.1103/PhysRevB.26.6355.
Hong J.I., Parker F.T., Solomon V.C., Madras P., Smith D.J., Berkowitz A.E. Fabrication of spherical particles with mixed amorphous/crystalline nanostructured cores and insulating oxide shells. Journal of Materials Research, 2008, vol. 23, no. 06, pp. 1758-1763. doi: https://doi.org/10.1557/JMR.2008.0199.
Wang W., Zhu F., Weng J., Xiao J., Lai W. Nanoparticle morphology in a granular Cu–Co alloy with giant magnetoresistance. Applied Physics Letters, 1998, vol. 72, no. 9, pp. 1118-1120. doi: https://doi.org/10.1063/1.120942.
Berkowitz A.E., Hansen M.F., Parker F.T., Vecchio K.S., Spada F.E., Lavernia E.J., Rodriguez R. Amorphous soft magnetic particles produced by spark erosion. Journal of Magnetism and Magnetic Materials, 2003, vol. 254-255, pp. 1-6. doi: https://doi.org/10.1016/S0304-8853(02)00932-0.
Kolbasov G.Ya., Ustinov A.I., Shcherba A.A., Perekos A.Ye., Danilov M.O., Vyunova N.V., Zakharchenko S.N., Hossbah G. Application of volumetric electric-spark dispersion for the fabrication of Ti-Zr-Ni hydrogen storage alloys. Journal of Power Sources, 2005, vol. 150, pp. 276-281. doi: https://doi.org/10.1016/j.jpowsour.2005.02.025.
Jin C.H., Si P.Z., Xiao X.F., Feng H., Wu Q., Ge H.L., Zhong M. Structure and magnetic properties of Cr/Cr2O3/CrO2 microspheres prepared by spark erosion and oxidation under high pressure of oxygen. Materials Letters, 2013. vol. 92, pp. 213-215. doi: https://doi.org/10.1016/j.matlet.2012.10.126.
Harrington T., McElfresh C., Vecchio K.S. Spark erosion as a high-throughput method for producing bimodal nanostructured 316L stainless steel powder. Powder Technology, 2018, vol. 328, pp. 156-166. doi: https://doi.org/10.1016/j.powtec.2018.01.012.
Berkowitz A.E., Walter J.L. Spark Erosion: A Method for Producing Rapidly Quenched Fine Powders. Journal of Materials Research, 1987, no. 2. pp. 277-288. doi: https://doi.org/10.1557/JMR.1987.0277.
Carrey J., Radousky H.B., Berkowitz A.E. Spark-eroded particles: influence of processing parameters. Journal of Applied Physics, 2004, vol. 95, no. 3, pp. 823-829. doi: https://doi.org/10.1063/1.1635973.
Shen B., Inoue A. Fabrication of large-size Fe-based glassy cores with good soft magnetic properties by spark plasma sintering. Journal of Materials Research, 2003, vol. 18, no. 9, pp. 2115-2121. doi: https://doi.org/10.1557/jmr.2003.0297.
Shydlovska N.A., Zakharchenko S.M., Zakharchenko M.F., Mazurenko I.L., Kulida M.A. Physical and Technical-economic Aspects of Modern Methods of Water Treatment for Thermal and Nuclear Power Engineering. Technical Electrodynamics, 2022, no. 4, pp. 69-77. (Ukr). doi: https://doi.org/10.15407/techned2022.04.069.
Goncharuk V.V., Shcherba A.A., Zakharchenko S.N., Savluk O.S., Potapchenko N.G., Kosinova V.N. Disinfectant action of the volume electrospark discharges in water. Khimiia i tehnologiia vody, 1999, vol. 21, no. 3, pp. 328-336. (Rus).
Shcherba A.A., Zakharchenko S.N., Lopatko K.G., Aftandilyants E.G. Application of volume electric spark dispersion for production steady to sedimentation hydrosols of biological active metals. Pratsi Instytutu Elektrodynamiky Natsionalnoi Akademii Nauk Ukrainy, 2009, no. 22, pp. 74-79. (Rus).
Zakharchenko S.M., Shydlovska N.A., Perekos A.O., Lopatko K.G., Savluk O.S. Features of Obtaining of Plasma-Erosion Nanodispersed Silver Hydrosols and Their Bactericidal and Fungicidal Properties. Metallofizika i Noveishie Tekhnologii, 2020, vol. 42, no. 6, pp. 829-851. (Rus). doi: https://doi.org/10.15407/mfint.42.06.0829.
Batsmanova L., Taran N., Konotop Ye., Kalenska S., Novytska N. Use of a Colloidal Solutions of Metal and Metal Oxide-Containing Nanoparticles as Fertilizer for Increasing Soybean Productivity. Journal of Central European Agriculture, 2020, vol. 21, no. 2, pp. 311-319. doi: https://doi.org/10.5513/JCEA01/21.2.2414.
Youssef F.S., El-Banna H.A., Elzorba H.Y., Gabal A.M. Application of Some Nanoparticles in the Field of Veterinary Medicine. International Journal of Veterinary Science and Medicine, 2019, vol. 7, no. 1. pp. 78-93. doi: https://doi.org/10.1080/23144599.2019.1691379.
Shydlovska N.A., Zakharchenko S.M., Cherkaskyi O.P. Physical Prerequisites of Construction of Mathematical Models of Electric Resistance of Plasma-erosive Loads. Technical Electrodynamics, 2017, no 2, pp. 5-12. (Ukr) doi: https://doi.org/10.15407/techned2017.02.005.
Raizer Yu.P. Gas Discharge Physics. Berlin, Springer, 1991. 449 p.
Lo A., Cessou A., Lacour C., Lecordier B., Boubert P., Xu D., Laux C.O., Vervisch P. Streamer-to-spark transition initiated by a nanosecond overvoltage pulsed discharge in air. Plasma Sources Science and Technology, 2017, vol. 26, no. 4. art. no. 045012. doi: https://doi.org/10.1088/1361-6595/aa5c78.
Shcherba A.A., Zakharchenko S.N., Yatsyuk S.A., Kucheryavaya I.N., Lopatko K.G., Aftandilyants E.G. Analysis of the Methods of Increasing the Efficiency of Electric-erosive Coagulation During Cleaning of Aqueous Media. Technical Electrodynamics. Thematic Issue «Power Electronics and Energy Efficiency», 2008, part 2, pp. 120-125. (Rus).
Shcherba A.A., Zakharchenko S.N., Suprunovskaya N.I., Shevchenko N.I. The influence of repetition rate of discharge pulses on electrical resistance of current-conducting granular layer during its electric-spark treatment. Technical Electrodynamics, 2006, no. 2, pp. 10-14.
Shcherba A.A., Suprunovska N.І., Ivashchenko D.S. Determination of Probabilistic Properties of Electrical Characteristics of Circuits of Electric Discharge Installations Taking into Account their Stochastically Changing Parameters. Technical Electrodynamics, 2019, no. 4, pp. 3-11. (Rus). doi: https://doi.org/10.15407/techned2019.04.003.
Shuaibov O.K., Malinina A.O., Hrytsak R.V., Malinin O.M., Bilak Yu.Yu., Gomoki Z.T., Vatrala M.I. Characteristics and Parameters of Overstressed Nanosecond Discharge Plasma Between Copper Electrodes in Argon. Metallofizika i Noveishie Tekhnologii, 2021, vol. 43, no. 12. pp. 1683-1706. (Ukr). doi: https://doi.org/10.15407/mfint.43.12.1683.
ToupTek. Download. Available at: https://www.touptek.com/download (Accessed 15 March 2023).
ToupTek. Download. Software Download. Help files for ToupCam camera. Available at: http://www.touptek.com/download/showdownload.php?lang=en&id=7 (Accessed 15 March 2023).
Medvedev N., Zastrau U., Forster E., Gericke D.O., Rethfeld B. Short-Time Electron Dynamics in Aluminum Excited by Femtosecond Extreme Ultraviolet Radiation. Physical Review Letters, 2011, vol. 107, art. no. 165003. doi: https://doi.org/10.1103/PhysRevLett.107.165003.
Kim S.J., Lee Y.S., Cho Ch.H., Choi M.S., Seong I.H., Lee J.J., Kim D.W., You Sh.J. Observation of prior light emission before arcing development in a low-temperature plasma with multiple snapshot analysis. Scientific Reports, 2022, no. 12, art. no. 20976. doi: https://doi.org/10.1038/s41598-022-25550-2.
Baranov M.I. A generalized physical principle of development of plasma channel of a high-voltage pulse spark discharge in a dielectric. Electrical Engineering & Electromechanics, 2024, no. 1, pp. 34-42. doi: https://doi.org/10.20998/2074-272X.2024.1.05.
Shcherba A.A., Shcherba M.A., Peretyatko Ju.V. Electro-Physical Processes of Degradation of Cross-Linked Polyethylene Insulation of Power Cables and Self-Carrying Insulated Wires under Non-Sinusoidal Voltages and Currents. Technical Electrodynamics, 2023, no. 1. pp. 3-6. (Ukr). doi: https://doi.org/10.15407/techned2023.01.03.
Bereka V.O., Bozhko I.V., Kondratenko I.P. Influence of Parameters of Water Movement at its Treatments on Energy Efficiency Pulse Barrier Discharge. Technical Electrodynamics, 2022, no. 3, pp. 62-68. (Ukr). doi: https://doi.org/10.15407/techned2022.03.062.
Zaidel’ A.N., Prokof’ev V.K., Raiskii S.M., Slavnyi V.A., Shreider E.Ya. Tables of Spectral Lines. New York, Springer, 1970. 782 p. doi: https://doi.org/10.1007/978-1-4757-1601-6.
Parigger C.G., Hornkohl J.O., Nemes L. Measurements of aluminum and hydrogen microplasma. Applied Optics, 2007, vol. 46, no. 19, pp. 4026-4031. doi: https://doi.org/10.1364/AO.46.004026.
Soo M., Goroshin S., Glumac N., Kumashiro K., Vickery J., Frost D.L., Bergthorson J.M. Emission and Laser Absorption Spectroscopy of Flat Flames in Aluminum Suspensions. Combustion and Flame, 2017, vol. 180, pp. 230-238. doi: https://doi.org/10.1016/j.combustflame.2017.03.006.
Sarvan M., Radić-Perić J., Kasalica B., Belča I., Stojadinović S., Perić M. Investigation of Long-duration Plasma Electrolytic Oxidation of Aluminum by Means of Optical Spectroscopy. Surface & Coatings Technology, 2014, vol. 254, pp. 270-276. doi: https://doi.org/10.1016/j.surfcoat.2014.06.029.
Liu R., Wu J., Xue W., Qu Ya., Yang Ch., Wang B., Wu X. Discharge Behaviors During Plasma Electrolytic Oxidation on Aluminum Alloy. Materials Chemistry and Physics, 2014, vol. 148, no. 1-2, pp. 284-292. doi: https://doi.org/10.1016/j.matchemphys.2014.07.045.
Qayyum A., Akhtar M.N., Riffat T. Light Emission from Sputtered Aluminum Atoms and Ions Produced by Ion Bombardment. Radiation Physics and Chemistry, 2005, vol. 72, no. 6, pp. 663-667. doi: https://doi.org/10.1016/j.radphyschem.2004.05.048.
Stojadinovic S., Vasilic R., Petkovic M., Nedic Z., Kasalica B., Belca I., Zekovic Lj. Luminescence properties of oxide films formed by anodization of aluminum in 12-tungstophosphoric acid. Electrochimica Acta, 2010, vol. 55, no. 12, pp. 3857-3863. doi: https://doi.org/10.1016/j.electacta.2010.02.011.
Staninski K., Piskuła Z., Kaczmarek M. Photo- and electroluminescence properties of lanthanide tungstatedoped porous anodic aluminum oxide. Optical Materials, 2017, vol. 64, pp. 142-146. doi: https://doi.org/10.1016/j.optmat.2016.12.003.
Stepniowski W.J., Norek M., Michalska-Domanska M., Bombalska A., Nowak-Stepniowska A., Kwasny M., Bojar Z. Fabrication of Anodic Aluminum Oxide with Incorporated Chromate Ions. Applied Surface Science, 2012, vol. 259, pp. 324-330. doi: https://doi.org/10.1016/j.apsusc.2012.07.043.
Shcherba A.A, Shtompel I.V. Analysis electrical parameters and dynamics of spark discharges in a layer of current-conducting granules. Stabilizatsiia parametrov elektricheskoi energii, 1991, pp. 65-74. (Rus).
Abcibc.com. Light wavelengths. Available at: http://abcibc.com/photo-reference-tables.php?art=19 (Accessed 15 March 2023). (Rus).
Nijdam S., Teunissen J., Ebert U. The physics of streamer discharge phenomena. Plasma Sources Science and Technology, 2020, vol. 29, no. 10, art. no. 103001. doi: https://doi.org/10.1088/1361-6595/abaa05.
Ninyovskij V., Murmantsev A., Veklich A., Boretskij V. Plasma spectroscopy of electric spark discharge between silver granules immersed in water. Energetika, 2022, vol. 68, no. 1, pp. 107-114. doi: https://doi.org/10.6001/energetika.v68i1.4862.
Veklich A., Tmenova T., Zazimko O., Trach V., Lopatko K., Titova L., Boretskij V., Aftandiliants Ye., Lopatko S., Rogovskiy I. Regulation of Biological Processes with Complexions of Metals Produced by Underwater Spark Discharge. Nanooptics and Photonics, Nanochemistry and Nanobiotechnology and Their Applications: Selected Proceedings of the 7th International Conference Nanotechnology and Nanomaterials (NANO2019), pp. 283-306. doi: https://doi.org/10.1007/978-3-030-52268-1_23.
Aurora. Available at: https://en.wikipedia.org/wiki/Aurora (Accessed 15 March 2023).
Shydlovska N.A., Zakharchenko S.M., Cherkassky O.P. The Analysis of Electromagnetic Processes in Output Circuit of the Generator of Discharge Pulses with Non-linear Model of Plasma-erosive Load at Change Their Parameters in Wide Ranges. Technical Electrodynamics, 2016, no. 1. pp. 87-95. (Rus). doi: https://doi.org/10.15407/techned2016.01.087.
Zakharchenkо S.M., Perekos А.O., Shydlovska N.A., Ustinov A.I., Bоytsоv О.F., Vоynаsh V.Z. Electrospark Dispersion of Metal Materials. I. Influence of Velocity of Flow of Operating Fluid on Dispersity of Powders. Metallofizika i Noveishie Tekhnologii, 2018, vol. 40, no. 3, pp. 339-357 (Rus). doi: https://doi.org/10.15407/mfint.40.03.0339.
Murmantsev A., Veklich A., Boretskij V. Optical emission spectroscopy of plasma of electric spark discharge between metal granules in liquid. Applied Nanoscience, 2023, vol. 13, no. 7, pp. 5231-5237. doi: https://doi.org/10.1007/s13204-022-02744-8.
Tmenova T.A., Veklich A.N., Boretskij V.F., Cressault Y., Valensi F., Lopatko K.G., Aftandilyants Y.G. Optical Emission Spectroscopy of Plasma Underwater Electric Spark Discharges Between Metal Granules. Problems of Atomic Science and Technology, 2017, vol. 107, no. 1, pp. 132-135.
Li S.-Y., Li S.-C., Sui L.-Z., Jiang Y.-F., Chen A.-M., Jin M.-X. Contribution of nitrogen atoms and ions to the luminescence emission during femotosecond filamentation in air. Physical Review A, 2016, vol. 93, no. 1, art. no. 013405. doi: https://doi.org/10.1103/PhysRevA.93.013405.
Lianzhu Z., Shuxia Z., Xiulan M. Characterization of Nitrogen Glow Discharge Plasma via Optical Emission Spectrum Simulation. Plasma Science and Technology, 2008, vol. 10, no. 4, pp. 455-462. doi: https://doi.org/10.1088/1009-0630/10/4/11.
Wang Z., Cohen S.A., Ruzic D.N., Goeckner M.J. Nitrogen atom energy distributions in a hollow-cathode planar sputtering magnetron. Physical Review E, 2000, vol. 61, no. 2, pp. 1904-1911. doi: https://doi.org/10.1103/PhysRevE.61.1904.
Bogaerts A., Gijbels R., Vlcek J. Modeling of glow discharge optical emission spectrometry: Calculation of the argon atomic optical emission spectrum. Spectrochimica Acta Part B: Atomic Spectroscopy, 1998, vol. 53, no. 11, pp. 1517-1526. doi: https://doi.org/10.1016/S0584-8547(98)00139-6.
Saloman E.B. Energy Levels and Observed Spectral Lines of Ionized Argon, Ar II through Ar XVIII. Journal of Physical and Chemical Reference Data, 2010, vol. 39, no. 3, art. no. 033101. doi: https://doi.org/10.1063/1.3337661.
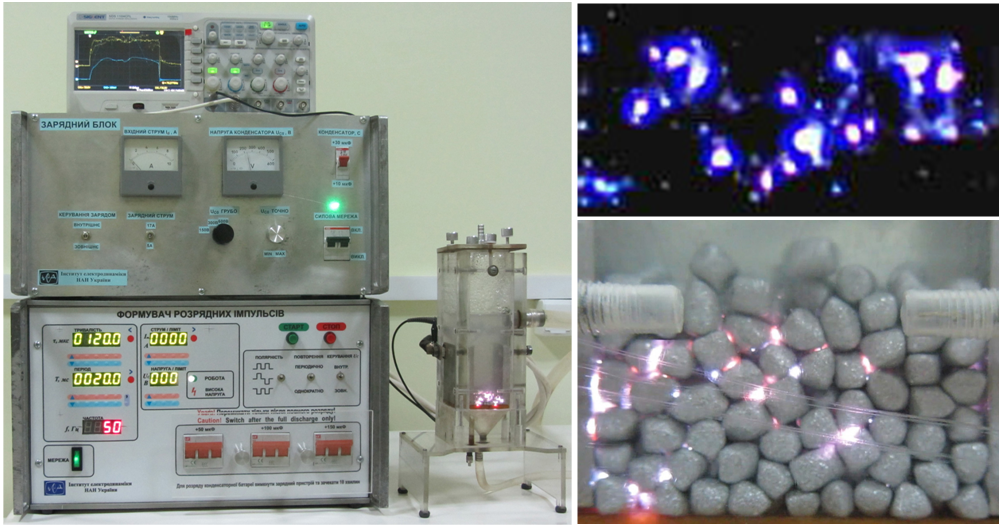
Downloads
Published
How to Cite
Issue
Section
License
Copyright (c) 2024 N. A. Shydlovska, S. M. Zakharchenko, M. F. Zakharchenko, M. A. Kulida, S. A. Zakusilo

This work is licensed under a Creative Commons Attribution-NonCommercial 4.0 International License.
Authors who publish with this journal agree to the following terms:
1. Authors retain copyright and grant the journal right of first publication with the work simultaneously licensed under a Creative Commons Attribution License that allows others to share the work with an acknowledgement of the work's authorship and initial publication in this journal.
2. Authors are able to enter into separate, additional contractual arrangements for the non-exclusive distribution of the journal's published version of the work (e.g., post it to an institutional repository or publish it in a book), with an acknowledgement of its initial publication in this journal.
3. Authors are permitted and encouraged to post their work online (e.g., in institutional repositories or on their website) prior to and during the submission process, as it can lead to productive exchanges, as well as earlier and greater citation of published work.