Modeling and analysis of electro-thermal processes in installations for induction heat treatment of aluminum cores of power cables
DOI:
https://doi.org/10.20998/2074-272X.2024.1.07Keywords:
electromagnetic processes, induction heat treatment, aluminum conductive core, power cables, multiscale modeling, current frequency, inductor efficiencyAbstract
Introduction. The development of the electric power industry is directly related to the improvement of cable lines. Cable lines meet modern requirements for reliability, they are increasingly used. Problem. Currently, power cables with an aluminum multi-conductor core, which requires heat treatment - an annealing process at the stage of the technological manufacturing process, are widespread. This process makes it possible to desirably reduce the electrical resistance of the wire and increase its flexibility. For effective use of induction heating during annealing of an aluminum core, it is necessary to determine the optimal frequency of the power source of the inductor. Considering the long length of the inductor and the large number of its turns, the numerical calculation of the electromagnetic field, which is necessary for calculating the equivalent electrical parameters of the turns of the inductor and its efficiency, requires significant computer resources. The goal is to develop a computer model for calculating electro-thermal processes in an induction plant for heating (up to the annealing temperature) an aluminum core of a power cable moving in the magnetic field of a long multi-turn inductor, as well as obtaining frequency dependences of the equivalent R, L parameters of such an inductor and determining the optimal the value of the frequency of the power source, which corresponds to the maximum value of the electrical efficiency of the inductor. Methodology. The mathematical model was developed to analyze the coupled electromagnetic and thermal processes occurring in a core moving in a time-harmonic magnetic field of an inductor at a constant speed. The differential equations for the electromagnetic and temperature fields, taking into account the boundary conditions, represent a coupled electro-thermal problem that was solved numerically by the finite element method using the Comsol software package. For a detailed analysis of the electromagnetic processes in the inductor, an additional problem was considered at the level of the elementary cell, which includes one turn of the inductor and a fragment of the core located near this turn. Results. According to the results of the calculation of the electromagnetic field in the area of the elementary cell, the equivalent electrical parameters of one turn of the inductor and the entire multi-turn inductor were calculated depending on the frequency of the electric current. The frequency dependences of the electrical efficiency of the inductor were calculated. Originality. Taking into account the design features of the inductor (its long length and large number of turns), the method of multiscale modeling was used. Electro-thermal processes in the core were studied at the macro level, and the distribution of the electromagnetic field and electric current density in the cross-section of the massive copper turn of the inductor was calculated at the micro level – at the level of an elementary cell containing only one turn of the inductor. The frequency dependences of the equivalent R, L parameters of the inductor, taking into account the skin effect, the proximity effect, and the geometric effect, were obtained, and the quantitative influence of the electric current frequency on these effects was studied. Practical value. The dependence of the electrical efficiency of the inductor on the frequency of the power source was obtained and it was shown that for effective heating of an aluminum core with a diameter of 28 mm, the optimal value of the frequency is in the range of 1–2 kHz, and at the same time the electrical efficiency reaches values of ηind = 0.3–0.33, respectively.
References
Kyrylenko O.V., Zhuikov V.Y., Denysiuk S.P. Use of dynamic tarification for optimization microgrid technical and economic indicators in local electricity markets. Technical Electrodynamics, 2022, no. 3, pp. 37-48. (Ukr). doi: https://doi.org/10.15407/techned2022.03.037.
Lezama F., Soares J., Hernandez-Leal P., Kaisers M., Pinto T., Vale Z. Local Energy Markets: Paving the Path Toward Fully Transactive Energy Systems. IEEE Transactions on Power Systems, 2019, vol. 34, no. 5, pp. 4081-4088. doi: https://doi.org/10.1109/TPWRS.2018.2833959.
Sinha A., Basu A.K., Lahiri R.N., Chowdhury S., Chowdhury S.P., Crossley P.A. Setting of Market Clearing Price (MCP) in Microgrid Power Scenario. 2008 IEEE Power and Energy Society General Meeting - Conversion and Delivery of Electrical Energy in the 21st Century, 2008, pp. 1-8. doi: https://doi.org/10.1109/PES.2008.4596357.
Hirsch A., Parag Y., Guerrero J. Microgrids: A review of technologies, key drivers, and outstanding issues. Renewable and Sustainable Energy Reviews, 2018, vol. 90, pp. 402-411. doi: https://doi.org/10.1016/j.rser.2018.03.040.
Zolotarev V.M., Shcherba A.A., Karpushenko V.P., Peretiatko Yu.V., Zolotarev V.V., Oboznyi A.L. Recommendations for the selection of characteristics, designs and application of self-carrying insulated wires produced by PJSC YUZHCABLE WORKS for overhead power lines of increased reliability and safety. Kharkiv, Maidan Publ., 2008. 62 p. (Ukr).
Peschke E., Olshausen R. Cable Systems for High and Extra-High Voltage. MCD Verlag, 1999. 296 p.
Shydlovsky A.K., Shcherba A.A., Zolotariov V.M., Podoltsev A.D., Kucheriavaia I.N. Polymer insulated cables for extra high voltage. Kyiv, Institute of Electrodynamics National Academy of Sciences of Ukraine Publ., 2013. 550 p. (Rus).
Bezprozvannych G.V., Pushkar O.A. Increasing noise immunity of cables for fire protection systems. Electrical Engineering & Electromechanics, 2020, no. 4, pp. 54-58. doi: https://doi.org/10.20998/2074-272X.2020.4.07.
Kyrylenko O.V., Blinov I.V., Parus E.V., Trach I.V. Evaluation of efficiency of use of energy storage system in electric networks. Technical Electrodynamics, 2021, no. 4, pp. 44-54. (Ukr). doi: https://doi.org/10.15407/techned2021.04.044.
Nijman E., Buchegger B., Böhler E., Rejlek J. Experimental Characterization and Dynamic Modelling of Electrical Cables. SAE International Journal of Advances and Current Practices in Mobility, 2023, vol. 5, no. 2, pp. 888-896. doi: https://doi.org/10.4271/2022-01-0952.
Baranov M.I., Rudakov S.V. Electrothermal Action of the Pulse of the Current of a Short Artificial-Lightning Stroke on Test Specimens of Wires and Cables of Electric Power Objects. Journal of Engineering Physics and Thermophysics, 2018, vol. 91, no. 2, pp. 544-555. doi: https://doi.org/10.1007/s10891-018-1775-2.
Shazly J.H., Mostafa M.A., Ibrahim D.K., Abo El Zahab E.E. Thermal analysis of high‐voltage cables with several types of insulation for different configurations in the presence of harmonics. IET Generation, Transmission & Distribution, 2017, vol. 11, no. 14, pp. 3439-3448. doi: https://doi.org/10.1049/iet-gtd.2016.0862.
Shcherba A.A., Suprunovska N.I. Electric energy loss at energy exchange between capacitors as function of their initial voltages and capacitances ratio. Technical Electrodynamics, 2016, no. 3, pp. 9-11. doi: https://doi.org/10.15407/techned2016.03.009.
Beletsky O.A., Suprunovska N.I., Shcherba A.A. Dependences of power characteristics of circuit at charge of supercapacitors. Technical Electrodynamics, 2016, no. 1, pp. 3-10. (Ukr). doi: https://doi.org/10.15407/techned2016.01.003.
Ochin P., Gilchuk A.V., Monastyrsky G.E., Koval Y., Shcherba A.A., Zaharchenko S.N. Martensitic Transformation in Spark Plasma Sintered Compacts of Ni-Mn-Ga Powders Prepared by Spark Erosion Method in Cryogenic Liquids. Materials Science Forum, 2013, vol. 738-739, pp. 451-455. doi: https://doi.org/10.4028/www.scientific.net/MSF.738-739.451.
Vinnychenko D.V. Influence of electrical parameters of high-voltage electric-discharge systems for synthesis of nanocarbon on their performance and specific power inputs. Technical Electrodynamics, 2016, no. 4, pp. 95-97. (Ukr). doi: https://doi.org/10.15407/techned2016.04.095.
Ametani A., Xue H., Ohno T., Khalilhezhad H. Electromagnetic Transients in Large HV Cable Networks: Modeling and calculations. The Institute of Engineering Technology, 2021. 591 p. doi: https://doi.org/10.1049/PBPO204E.
Mokhort A.V., Chumak M.G. Thermal processing of metals. Kyiv, Lybid Publ., 2002. 512 p. (Ukr)
Rudnev V., Loveless D., Cook R. Handbook of induction heating. Boca Raton, CRS Press, 2017. 776 p. doi: https://doi.org/10.1201/9781315117485.
Sharma G.K., Pant P., Jain P.K., Kankar P.K., Tandon P. Numerical and experimental analysis of heat transfer in inductive conduction based wire metal deposition process. Proceedings of the Institution of Mechanical Engineers, Part C: Journal of Mechanical Engineering Science, 2022, vol. 236, no. 5, pp. 2395-2407. doi: https://doi.org/10.1177/09544062211028267.
Bao L., Wang B., You X., Li H., Gu Y., Liu W. Numerical and experimental research on localized induction heating process for hot stamping steel sheets. International Journal of Heat and Mass Transfer, 2020, vol. 151, art. no. 119422. doi: https://doi.org/10.1016/j.ijheatmasstransfer.2020.119422.
Mortimer J., Rudnev V., Clowes D., Shaw В. Intricacies of Induction Heating of Wires, Rods, Ropes & Cables. Wire Forming Technology International, Winter 2019, pр. 46-50.
Lope I., Acero J., Carretero C. Analysis and Optimization of the Efficiency of Induction Heating Applications With Litz-Wire Planar and Solenoidal Coils. IEEE Transactions on Power Electronics, 2016, vol. 31, no. 7, pp. 5089-5101. doi: https://doi.org/10.1109/TPEL.2015.2478075.
Fisk M., Ristinmaa M., Hultkrantz A., Lindgren L.-E. Coupled electromagnetic-thermal solution strategy for induction heating of ferromagnetic materials. Applied Mathematical Modelling, 2022, vol. 111, pp. 818-835. doi: https://doi.org/10.1016/j.apm.2022.07.009.
Siesing L., Frogner K., Cedell T., Andersson M. Investigation of Thermal Losses in a Soft Magnetic Composite Using Multiphysics Modelling and Coupled Material Properties in an Induction Heating Cell. Journal of Electromagnetic Analysis and Applications, 2016, vol. 08, no. 09, pp. 182-196. doi: https://doi.org/10.4236/jemaa.2016.89018.
Amarulloh A., Haikal H., Atmoko N.T., Utomo B.R., Setiadhi D., Marchant D., Zhu X., Riyadi T.W.B. Effect of power and diameter on temperature and frequency in induction heating process of AISI 4140 steel. Mechanical Engineering for Society and Industry, 2022, vol. 2, no. 1, pp. 26-34. doi: https://doi.org/10.31603/mesi.6782.
Shang F., Sekiya E., Nakayama Y. Application of High-Frequency Induction Heating Apparatus to Heat Treatment of 6061 Aluminum Alloy. Materials Transactions, 2011, vol. 52, no. 11, pp. 2052-2060. doi: https://doi.org/10.2320/matertrans.L-M2011825.
Podoltsev A.D., Kucheriavaia I.N. Multiscale modeling in electrical engineering. Kyiv, Institute of Electrodynamics National Academy of Sciences of Ukraine Publ., 2011. 255 p. (Rus).
Kovachki N., Liu B., Sun X., Zhou H., Bhattacharya K., Ortiz M., Stuart A. Multiscale modeling of materials: Computing, data science, uncertainty and goal-oriented optimization. Mechanics of Materials, 2022, vol. 165, art. no. 104156. doi: https://doi.org/10.1016/j.mechmat.2021.104156.
Ryu C.J., Kudeki E., Na D.-Y., Roth T.E., Chew W.C. Fourier Transform, Dirac Commutator, Energy Conservation, and Correspondence Principle for Electrical Engineers. IEEE Journal on Multiscale and Multiphysics Computational Techniques, 2022, vol. 7, pp. 69-83. doi: https://doi.org/10.1109/JMMCT.2022.3148215.
Available at: https://www.comsol.com (accessed 22 March 2023).
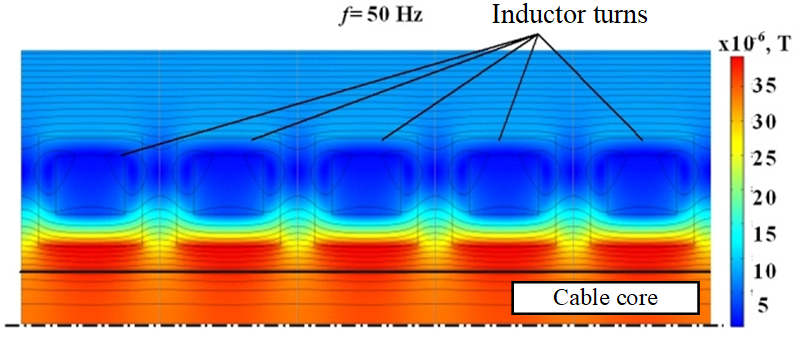
Downloads
Published
How to Cite
Issue
Section
License
Copyright (c) 2024 A. A. Shcherba, O. D. Podoltsev, N. I. Suprunovska, R. V. Bilianin, T. Yu. Antonets, I. M. Masluchenko

This work is licensed under a Creative Commons Attribution-NonCommercial 4.0 International License.
Authors who publish with this journal agree to the following terms:
1. Authors retain copyright and grant the journal right of first publication with the work simultaneously licensed under a Creative Commons Attribution License that allows others to share the work with an acknowledgement of the work's authorship and initial publication in this journal.
2. Authors are able to enter into separate, additional contractual arrangements for the non-exclusive distribution of the journal's published version of the work (e.g., post it to an institutional repository or publish it in a book), with an acknowledgement of its initial publication in this journal.
3. Authors are permitted and encouraged to post their work online (e.g., in institutional repositories or on their website) prior to and during the submission process, as it can lead to productive exchanges, as well as earlier and greater citation of published work.