New design and comparative study via two techniques for wind energy conversion system
DOI:
https://doi.org/10.20998/2074-272X.2021.3.03Keywords:
synergetic controller, sliding mode controller, maximum power point tracking, macro-variable, wind energy conversion systemAbstract
Introduction. With the advancements in the variable speed direct drive design and control of wind energy systems, the efficiency and energy capture of these systems is also increasing. As such, numerous linear controllers have also been developed, in literature, for MPPT which use the linear characteristics of the wind turbine system. The major limitation in all of those linear controllers is that they use the linearized model and they cannot deal with the nonlinear dynamics of a system. However, real systems exhibit nonlinear dynamics and a nonlinear controller is required to handle such nonlinearities in real-world systems. The novelty of the proposed work consists in the development of a robust nonlinear controller to ensure maximum power point tracking by handling nonlinearities of a system and making it robust against changing environmental conditions. Purpose. In the beginning, sliding mode control has been considered as one of the most powerful control techniques, this is due to the simplicity of its implementation and robustness compared to uncertainties of the system and external disturbances. Unfortunately, this type of controller suffers from a major disadvantage, that is, the phenomenon of chattering. Methods. So in this paper and in order to eliminate this phenomenon, a novel non-linear control algorithm based on a synergetic controller is proposed. The objective of this control is to maximize the power extraction of a variable speed wind energy conversion system compared to sliding mode control by eliminating the phenomenon of chattering and have a good power quality by fixing the power coefficient at its maximum value and the Tip Speed Ratio maintained at its optimum value. Results. The performance of the proposed nonlinear controllers has been validated in MATLAB/Simulink environment. The simulation results show the effectiveness of the proposed scheme, suppression of the chattering phenomenon and robustness of the proposed controller compared to the sliding mode control law.
References
Jeong H., Lee K., Choi S., W. Choi. Performance improvement of LCL-filter-based grid-connected inverters using PQR power transformation. IEEE Transactions on Power Electronics, 2010, vol. 25, no. 5, pp. 1320-1330. doi: https://doi.org/10.1109/tpel.2009.2037225.
Lee J.-S., Jeong H.-G., Lee K.-B. Active damping for wind power systems with LCL filters using a DFT. Journal of Power Electronics, 2012, vol. 12, no. 2, pp. 326-332. doi: https://doi.org/10.6113/jpe.2012.12.2.326.
Bounar N., Labdai S., Boulkroune A., Farza M., M’Saad M. Adaptive fuzzy control scheme for variable-speed wind turbines based on a doubly-fed induction generator. Iranian Journal of Science and Technology, Transactions of Electrical Engineering, 2020, vol. 44, no. 2, 629-641. doi: https://doi.org/10.1007/s40998-019-00276-6.
Agarwal V., Aggarwal R.K., Patidar P., Patki C. A novel scheme for rapid tracking of maximum power point in wind energy generation systems. IEEE Transactions on Energy Conversion, 2010, vol. 25, no. 1, pp. 228-236. doi: https://doi.org/10.1109/tec.2009.2032613.
Rolan A., Luna A., Vazquez G., Aguilar D., Azevedo G. Modeling of a variable speed wind turbine with a permanent magnet synchronous generator. 2009 IEEE International Symposium on Industrial Electronics, 2009, pp. 734-739. doi: https://doi.org/10.1109/isie.2009.5218120.
Chan T., Lai L.L. Permanent-magnet machines for distributed power generation: a review. 2007 IEEE Power Engineering Society General Meeting, 2007, pp. 1-6. doi: https://doi.org/10.1109/PES.2007.385575.
Jlassi I., Estima J.O., Khojet El Khil S., Mrabet Bellaaj N., Marques Cardoso A.J. Multiple open-circuit faults diagnosis in back-to-back converters of PMSG drives for wind turbine systems. IEEE Transactions on Power Electronics, 2015, vol. 30, no. 5, pp. 2689-2702. doi: https://doi.org/10.1109/TPEL.2014.2342506.
Bouderbala M., Bossoufi B., Lagrioui A., Taoussi M., Aroussi H.A., Ihedrane Y. Direct and indirect vector control of a doubly fed induction generator based in a wind energy conversion system. International Journal of Electrical and Computer Engineering, 2019, vol. 9, no. 3, pp. 1531-1540. doi: https://doi.org/10.11591/ijece.v9i3.pp1531-1540.
He Q., Zhang F., Liu D., Gao C., Shi S., Xi P. Analysis on the development status and problems of China's offshore wind power. 2018 2nd IEEE Conference on Energy Internet and Energy System Integration (EI2), 2018, pp. 1-4. doi: https://doi.org/10.1109/EI2.2018.8582177.
Worku M.Y., Abido M.A. Maximum Power Control of DFIG Based Grid Connected Wind Turbine Generator System. Renewable Energy and Power Quality Journal, 2018, vol. 1, pp. 444-449. doi: https://doi.org/10.24084/repqj16.344.
Boukhezzar B., Lupu L., Siguerdidjane H., Hand M. Multivariable control strategy for variable speed, variable pitch wind turbines. Renewable Energy, 2007, vol. 32, no. 8, pp. 1273-1287. doi: https://doi.org/10.1016/j.renene.2006.06.010.
Wang Q., Chang L. An intelligent maximum power extraction algorithm for inverter-based variable speed wind turbine systems. IEEE Transactions on Power Electronics, 2004, vol. 19, no. 5, pp. 1242-1249. doi: https://doi.org/10.1109/TPEL.2004.833459.
Toulabi M., Bahrami S., Ranjbar A.M. An Input-to-State Stability Approach to Inertial Frequency Response Analysis of Doubly-Fed Induction Generator-Based Wind Turbines. IEEE Transactions on Energy Conversion, 2017, vol. 32, no. 4, pp. 1418-1431. doi: https://doi.org/10.1109/TEC.2017.2696510.
Kumar D., Chatterjee K. A review of conventional and advanced MPPT algorithms for wind energy systems. Renewable and Sustainable Energy Reviews, 2016, vol. 55, pp. 957-970. doi: https://doi.org/10.1016/j.rser.2015.11.013.
Lee S.-W., Chun K.-H. Adaptive Sliding Mode Control for PMSG Wind Turbine Systems. Energies, 2019, vol. 12, no. 4, p. 595. doi: https://doi.org/10.3390/en12040595.
Li S., Haskew T.A., Xu L. Conventional and novel control designs for direct driven PMSG wind turbines. Electric Power Systems Research, 2010, vol. 80, no. 3, pp. 328-338. doi: https://doi.org/10.1016/j.epsr.2009.09.016.
Pratap B., Singh N., Kumar V. Robust Control of Variable Speed Wind Turbine Using Quasi-Sliding Mode Approach. Procedia Computer Science, 2018, vol. 125, pp. 398-404. doi: https://doi.org/10.1016/j.procs.2017.12.052.
Kolesnikov A., Veselov G., Kolesnikov A. Modern applied control theory: synergetic approach in control theory. Moscow-Taganrog, TRTU Publ., 2000.
Attoui H., Khaber F., Melhaoui M., Kassmi K., Essounbouli N. Development and experimentation of a new MPPT synergetic control for photovoltaic systems. Journal of Optoelectronics and Advanced Materials, 2016, vol. 18, no. 1-2, pp. 165-173. Available at: https://joam.inoe.ro/articles/development-and-experimentation-of-a-new-mppt-synergetic-control-for-photovoltaic-systems/ (accessed 21 May 2020).
Akoro E., Tevi G.J., Faye M.E., Doumbia M.L., Maiga A.S. Artificial Neural Network Photovoltaic Generator Maximum Power Point Tracking Method using Synergetic Control Algorithm. International Journal on Emerging Technologies, 2020, vol. 11, no. 2, pp. 590-594. Available at: https://www.researchtrend.net/ijet/pdf/Artificial%20Neural%20Network%20Photovoltaic%20Generator%20Maximum%20Power%20Point%20Tracking%20Method%20using%20Synergetic%20Control%20Algorithm%20Edjadessamam%20AKORO%201893n7.pdf (accessed 21 May 2020).
Ounnas D., Ramdani M., Chenikher S., Bouktir T. Optimal Reference Model Based Fuzzy Tracking Control for Wind Energy Conversion System. International Journal of Renewable Energy Research, 2016, vol. 6, no. 3, pp. 1129-1136. Available at: https://www.ijrer.org/ijrer/index.php/ijrer/article/view/4258/pdf (accessed 21 May 2020).
Aissou R., Rekioua T., Rekioua D., Tounzi A. Nonlinear predictive control of a permanent magnet synchronous generator used in wind energy. Journal of Electrical Engineering, 2014, vol. 14, no. 4, pp. 331-336. Available at: http://jee.ro/articles/WN1397935592W5352cde8e8fe5.pdf (accessed 21 May 2020).
Daghigh A., Javadi H., Torkaman H. Considering wind speed characteristics in the design of a coreless AFPM synchronous generator. International Journal of Renewable Energy Research, 2016, vol. 6, no. 1, pp. 263-270. Available at: https://www.ijrer.org/ijrer/index.php/ijrer/article/view/3135/pdf (accessed 21 May 2020).
Watson S., Moro A., Reis V., Baniotopoulos C., Barth S., Bartoli G., Bauer F., Boelman E., Bosse D., Cherubini A., Croce A., Fagiano L., Fontana M., Gambier A., Gkoumas K., Golightly C., Latour M.I., Jamieson P., Kaldellis J., Macdonald A., Murphy J., Muskulus M., Petrini F., Pigolotti L., Rasmussen F., Schild P., Schmehl R., Stavridou N., Tande J., Taylor N., Telsnig T., Wiser R. Future emerging technologies in the wind power sector: A European perspective. Renewable and Sustainable Energy Reviews, 2019, vol. 113, art. no. 109270. doi: https://doi.org/10.1016/j.rser.2019.109270.
Messaoud M., Abdessamed R. Modeling and optimization of wind turbine driving permanent magnet synchronous generator. Jordan Journal of Mechanical and Industrial Engineering, 2011, vol. 5, no. 6. Available at: http://jjmie.hu.edu.jo/files/v5n6/JJMIE%20-77-10.pdf (accessed 21 May 2020).
Errami Y., Ouassaid M., Maaroufi M. A performance comparison of a nonlinear and a linear control for grid connected PMSG wind energy conversion system. International Journal of Electrical Power & Energy Systems, 2015, vol. 68, pp. 180-194. doi: https://doi.org/10.1016/j.ijepes.2014.12.027.
Bennassar A., Banerjee S., Jamma M., Essalmi A., Akherraz M. Real time high performance of sliding mode controlled induction motor drives. Procedia Computer Science, 2018, vol. 132, pp. 971-982. doi: https://doi.org/10.1016/j.procs.2018.05.113.
Evangelista C., Valenciaga F., Puleston P. Active and reactive power control for wind turbine based on a MIMO 2-sliding mode algorithm with variable gains. IEEE Transactions on Energy Conversion, 2013, vol. 28, no. 3, pp. 682-689. doi: https://doi.org/10.1109/TEC.2013.2272244.
Krim Y., Abbes D., Krim S., Faouzi Mimouni M. Power management and second-order sliding mode control for standalone hybrid wind energy with battery energy storage system. Proceedings of the Institution of Mechanical Engineers, Part I: Journal of Systems and Control Engineering, 2018, vol. 232, no. 10, pp. 1389-1411. doi: https://doi.org/10.1177/0959651818784320.
Meng Z., Shao W., Tang J., Zhou H. Sliding-mode control based on index control law for MPPT in photovoltaic systems. CES Transactions on Electrical Machines and Systems, 2018, vol. 2, no. 3, pp. 303-311. doi: https://doi.org/10.30941/CESTEMS.2018.00038.
Chavira F., Ortega-Cisneros S., Rivera J. A novel sliding mode control scheme for a PMSG-based variable speed wind energy conversion system. Energies, 2017, vol. 10, no. 10, art. no. 1476. doi: https://doi.org/10.3390/en10101476.
Levron Y., Shmilovitz D. Maximum power point tracking employing sliding mode control. IEEE Transactions on Circuits and Systems I: Regular Papers, 2013, vol. 60, no. 3, pp. 724-732. doi: https://doi.org/10.1109/TCSI.2012.2215760.
Rajendran S., Jena D. Backstepping sliding mode control for variable speed wind turbine. 2014 Annual IEEE India Conference (INDICON), 2014, pp. 1-6. doi: https://doi.org/10.1109/INDICON.2014.7030634.
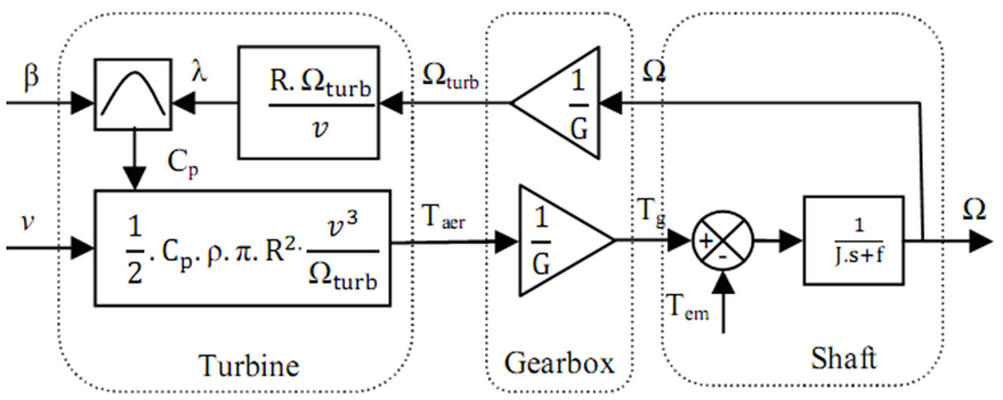
Downloads
Published
How to Cite
Issue
Section
License
Copyright (c) 2021 M.S. Mahgoun, A.E. Badoud

This work is licensed under a Creative Commons Attribution-NonCommercial 4.0 International License.
Authors who publish with this journal agree to the following terms:
1. Authors retain copyright and grant the journal right of first publication with the work simultaneously licensed under a Creative Commons Attribution License that allows others to share the work with an acknowledgement of the work's authorship and initial publication in this journal.
2. Authors are able to enter into separate, additional contractual arrangements for the non-exclusive distribution of the journal's published version of the work (e.g., post it to an institutional repository or publish it in a book), with an acknowledgement of its initial publication in this journal.
3. Authors are permitted and encouraged to post their work online (e.g., in institutional repositories or on their website) prior to and during the submission process, as it can lead to productive exchanges, as well as earlier and greater citation of published work.